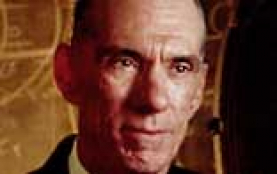
One of the most prominent psi research groups in recent decades was the Princeton Engineering Anomalies Research (PEAR) laboratory. It was founded in 1979 by Robert G Jahn of Princeton University to conduct research on the extended capacities of human consciousness. The program continued for nearly three decades, ending in 2007. As one of the highest ranking officers at an elite Ivy League university, Jahn was in a position to muster financial support and a rich academic context for potentially ground-breaking science. PEAR was a well-supported, technologically sophisticated effort to establish and explore anomalous effects of intention on physical systems, and anomalous communication of information across barriers of distance and time.
History
The PEAR lab was founded in 1979 by Robert G Jahn, then Dean of the School of Engineering and Applied Sciences at Princeton University. Jahn was a physicist and a professor in the Department of Mechanical and Aerospace Engineering, and also headed a NASA funded program researching electric propulsion systems for spacecraft. He was asked by an undergraduate to sponsor an independent study to try to replicate findings by Helmut Schmidt1 that suggested that the behaviour of sensitive equipment based on a physical random source could be influenced by human intention. The results of this study persuaded Jahn that the questions deserved a serious examination, using the best available protocols and technologies. He sought funding from private donors, and began building a staff and laboratory space. The first major financial support came from James MacDonnell of MacDonnell Douglas Aircraft Corporation, who thought it important to study possible effects of consciousness on sensitive instruments such as might be found in the emotionally intense environment of an aircraft cockpit.
Jahn attended professional conferences of specialists in psi research such as the Annual Convention of the Parapsychological Association, and at one of these he met developmental psychologist Brenda Dunne, who had presented her research on remote viewing. He recruited Dunne to help create a dedicated laboratory at Princeton University, and she accepted the position of Laboratory Manager for what became PEAR. Over the next few years the multi-disciplinary staff increased to include experimental psychologist Roger Nelson, electrical engineer John Bradish, astrophysicist York Dobyns, philosopher Arnold Lettieri and other researchers, as well as interns and students.
The PEAR lab produced a variety of publications documenting the research, including technical reports, peer-reviewed journal articles, and books. Jahn also worked with other mainstream scientists to create a broad-spectrum forum for science at the borders, called the Society for Scientific Exploration (SSE).
Experiments
The PEAR lab had from the beginning three areas of primary interest. Most of the experiments which were developed addressed mind-machine interaction (MMI) with a focus on the broad range of potential modulators of anomalous effects.2 The goal was to first establish that an anomalous effect of human intention could be demonstrated, and then to study what factors facilitated or hindered such effects. These factors specifically included both psychological and physical parameters.
The second experimental thrust was on remote viewing, which PEAR called ‘remote perception’ to reflect interest in multiple sensory modalities. The majority of trials used a precognitive protocol, where the perception was recorded before the target was selected, so the experiment was called Precognitive Remote Perception or PRP.
The third focus at PEAR was on modeling and theory building in an effort to develop an explanatory framework for empirical results that were difficult to integrate into standard scientific models.
Mind-Machine Interaction
The largest and longest running MMI experiment used a technologically sophisticated random event generator (REG) based on electron tunneling in solid state junctions. This quantum process is exploited to produce fundamentally unpredictable bit sequences. The design uses a ‘back-volted’ circuit where voltage pressure against the closed switch of a diode or transistor results in some electrons ‘tunneling’ through the forbidden gap. Because of quantum tunneling, a tiny, fundamentally unpredictable fluctuating voltage occurs after the junction; this is sampled and the high and low voltages are converted into a sequence of 1 and 0 binary digits or bits. Conceptually, such an REG (which is also called an RNG or random number generator) is equivalent to a high-speed electronic coin flipper. Modern technology is applied to ensure an output that meets statistical criteria for true randomness, which is tested in repeated calibration trials.
These true random number generators are to be distinguished from algorithmic pseudorandom number generators using computer software to create long sequences which look random, but are deterministic. A subset of PEAR REG experiments asked whether both types of random source would show anomalous consciousness-related effects, and tentatively concluded that the deterministic sources were not susceptible to the effects of intention. True random sources are labile, so the future state of a data sequence is in principle changeable. Other researchers have reported some MMI effects using pseudorandom sources so the question is not settled.
Random Event Generator (REG)
The REG experiment at PEAR typically used trials which were the sum of 200 bits drawn from the sequence at a rate of one trial per second. The bit generation rate was several thousand bits per second, so the experiment could explore the effect of larger trials (for instance 2,000 bits) as well as other physical parameters including the rate of bit or trial generation. The original REG device had switches to set such parameters to allow exploration. Later generations of the experiment used simpler, miniaturized random sources, with software to control the conditions which the PEAR program assessed.
Over many years, the REG experiment accumulated substantial evidence for an effect of human intention using a tri-polar protocol where operators (PEAR’s preferred name for subjects) succeeded overall in achieving high trial values under that instruction, low numbers when so instructed, and no significant deviation from expectation for baseline trials. In the original ‘benchmark’ REG experiment, the difference between high and low conditions in 2.5 million trials over twelve years of efforts by 91 operators is small but highly significant with a Z-score of 3.99 (~ 4 sigma) and a corresponding probability of 7 x 10-5 or odds of about 15,000 to 1 against chance as an explanation. Various parameters, such as the speed of data generation, the size of the trials, random vs volitional choice of intention, pairs of operators vs individuals were examined. Most had small effects, but of special interest was the effect size for bonded pairs – their scoring levels were significantly higher than for either of the individuals.
When all variations on the basic experiment are combined, producing a much larger database, the Z-score is 6.06 (6 sigma), and the probability is 6 x 10-10. Though the possibility these results are due to chance is virtually excluded, this represents a very small effect size of only a few parts in 10,000. But the finding has outsize implications given the highly controlled experiments because it does not fit easily into standard models of physics or psychology. A valid demonstration of mind-machine effects at any level requires serious consideration by physicists and, because human consciousness is involved, also by psychologists.3
The PEAR REG experiments were themselves conceptual replications of work by Helmut Schmidt and others, and over the past few decades many other laboratories did similar experiments, sometimes successfully replicating the anomalous results. A notable exception was a replication attempt by a consortium of three labs including PEAR and research groups in Giessen and Freiburg, Germany. This was a strict replication in the sense that PEAR software and REGs were used, and all three labs followed the same protocols. The results showed positive but non-significant trends at all three labs, despite what seemed to be suitable conditions and sufficient statistical power. Analysis showed significant deviations in various parameters, but in the primary replication measure, this large experiment failed to replicate.4 Nevertheless, meta-analyses over all known REG experiments including this one provide clear and highly significant evidence that human consciousness and intention can affect the behavior of random number generators.5
FieldREG
After more than a decade of laboratory research with REGs, the PEAR lab took advantage of advances in electronics and computer technology to miniaturize the random sources to take the experiments into the field. A new experiment called ‘FieldREG’ assessed data produced in environments which were conducive to subjective resonance or coherence such as small intimate groups, group rituals, sacred sites, and musical and theatrical performances. The expectation or hypothesis was that the coherent attention, even without explicit intention, would affect the behavior of the REG, producing anomalous deviations of the mean. Since this was not a directional prediction (no intention) the measure chosen was variance of the mean; strong deviations in either direction would indicate an anomalous effect.
Several exploratory datasets were collected under strict protocols in a replication paradigm where the same procedures were used in a variety of venues. The data collected in resonant/coherent venues conducive to group consciousness showed larger deviations than those generated in more mundane venues such as shopping centers, academic conferences, and business meetings. The composite results of the formal replications strongly confirm the general hypothesis, yielding a composite probability against chance for the resonant subset of p = 2.2 x10-6 compared to p = 0.91 for the mundane subset.6
Other Machines
Several other experiments asked the same basic question: Can human intention affect the behavior of well-calibrated physical devices and instruments? These included several devices that looked at micro-PK, that is, mental influences on a microscopic level, engaging quantum level variations in physical systems like the electronic RNG. Another example of this micro-PK category was the PEAR CHIP experiment. It was quite directly representative of the motivating questions about human intentions or emotions in a sensitive electronic environment. The experimental device was built around a widely used electronic component, a shift-register, which was supplied with voltage lower than its normally specified level, set and maintained precisely at the threshold where it might begin to make errors. The psi task was to increase or decrease the error rate or let it be, in the standard tripolar protocol, and as in the REG experiment, operators succeeded in generating a small effect in the direction of intention.7
At the other end of the dimensional scale, PEAR created a large machine which was formally called the Random Mechanical Cascade (RMC). The device had various nicknames and it was familiarly called Murphy, a name which arose from its complexity which ensured that it followed Murphy's Law: ‘If anything can go wrong, it will.’ It was nearly ten feet (~ three meters) tall and six feet (~ two meters) wide, with a Plexiglas front enclosing a matrix of 336 equally spaced nylon pins about ¾ inch in diameter (~ two cm). A conveyor belt brought 9,000 ¾ inch diameter polystyrene balls to the top where they dropped from a central funnel into the pin matrix where they bounced left and right through the pins.
The machine was modeled after a statistical demonstration display in a technology museum, where the bouncing balls gradually built up a Gaussian or bell curve distribution. The PEAR machine was instrumented to count the balls as they fell into nineteen collecting bins, with the data registered in computer files for analysis. Operators sitting across the room attempted to shift the distribution to the left or right or let the machine do a baseline run. The analysis controlled for physical changes such as component wear or effects of humidity by calculating differences between conditions within a tripolar set which would be completed typically within about 45 minutes.
The overall results showed a statistically significant difference between the right vs left conditions, with t = 3.89 and p < 10-4, or odds of about 10,000 to 1 against chance fluctuation.8 It is worth noting that although this experiment at first glance appears to be macroscopic, involving large forces, careful study shows it to actually involve only non-energetic decisions – does the ball bounce left or right?
PEAR also built a Linear Pendulum experiment, in which operators attempted to change the damping rate of a pendulum inside a Plexiglas box. The pendulum bob was a 2.5 inch quartz crystal ball suspended from precision bearings on a thirty-inch rod made of fused silica, which has a zero coefficient of thermal expansion. The speed of the swinging pendulum was measured by a fifty-nanosecond clock interrupted by a diode beam cut by a razor edge mounted on the pendulum rod. The high-quality bearings ensured that most of the damping forces were from passage through the air, and the change in damping rate was too small to see visually, but the precision instrumentation allowed measurement of changes with five-digit accuracy.
Again, data collection in the tripolar protocol allowed experimental control over changing environmental conditions including variations in barometric pressure. The overall result was a significant positive effect aligned with the operators’ intentions.9
Other experiments covered a wide range of physical phenomena. These included an exquisitely sensitive Fabry-Perot interferomenter (which created optical interference patterns), and a controlled miniature fountain displaying the delicate transition from laminar (smooth) to turbulent flow.
A dual-thermistor experiment asked whether operators could differentially change the readings of two sensitive heat detectors in the same controlled environment.
A small mobile robot whose direction and excursion distance was controlled by an REG traveled in a random path on a round table surface. The operator task was to mentally attract the robot or push it away. It was a playful experiment which operators enjoyed, and which showed modest but variable success corresponding to the assigned intentions. As a side note, the PEAR lab occasionally hosted visits from school classes. The students, about ten years old, were delighted by all the experiments they tried, but the mobile robot was their favorite. While PEAR did not conduct formal experiments with minor children, they appeared to be more successful than adults.
Several more physical experiments were built, some of which could be brought to completion and used in formal tests. Some did not make it to that stage because their sensitivity to environmental conditions made a completely clean, controlled experiment impractical. What is of special note is that all of these widely differing physical systems appeared to be susceptible to modulation correlated with human intention. The MMI experiments provide clear evidence that there is correlation of mental states with unexpected structure in data from a variety of physical systems characterized by a fundamental random aspect. To compare effect sizes in experiments with varied basic trial units an analogue to the ordinary calculation (Z/N-2) was developed which normalizes the Z-score by the square root of the number hours spent generating the effects. Using this metric, PEAR found the effect size was similar in all the MMI experiments.10
Secondary Parameters
The primary correlate in all the REG experiments was intention, but there are several secondary correlates or modulators.11 Individuals exhibited different levels and styles of performance (which PEAR called signatures) and there was suggestive evidence that the signature was similar in different experiments. A comprehensive analysis indicated that about 15% of the unselected operators were successful in the experimental tasks. Both random assignment and volitional selection of intentions had similar levels of achievement overall, but for some individuals, this modulator produced significant differences. Increasing the size of trials from twenty to 200 to 2,000 bits showed a modest increase of scoring level, but going to a two million bit trial size gave confounding results with a highly significant backwards effect – the high and low outcomes were reversed relative to operator intention.12
PEAR also assessed manual vs automatic trial sequencing in groups of fifty or 1,000 trials. Performance in this parameter depended on the individual operators. Most preferred the automatic sequencing, but interestingly, the largest effect size in the PEAR REG database was achieved by an operator who chose to use only the manual mode, explaining this allowed a meditative approach to the task.
Most experiments targeted the distribution mean, but a small subset used distribution variance as the target, and these also showed successful performance. One of the most important parameter variations PEAR tested was spatial and temporal separation of the operator from the REG device. Programs to collect data continuously enabled trials to be identified in an index, with intention periods of fifteen minutes (1,000 trials) marked, while the operator was at a distant location. The order and timing of High, Low, and Baseline intentions were communicated to the PEAR staff, who entered the information in the computer index, after which analysis could be completed. These remote trials not only showed successful performance, but slightly higher effect sizes, on average, compared with on-time, local trials.13
Remote Perception
Objective Analysis
The second experimental program at PEAR was initially a conceptual replication of so-called remote viewing (RV) experiments originated in the Stanford Research Institute (SRI) laboratory and described by Charles Tart, Russell Targ and Harold Puthoff.14 At PEAR, the focus of what were called Remote Perception, and later Precognitive Remote Perception (PRP) experiments, quickly shifted from simple demonstration to sophisticated measurement. Originally, the efficacy of the remote perception was determined by human judging – rating a set of targets including the actual target and several decoys. PEAR replaced this with a protocol where the ‘agent’ at the scene filled out a binary descriptor list indicating whether each of thirty elements were present or absent from the scene. The ‘percipient’ encoded his or her experience in a narrative and sketches, but also using the same descriptor list, and the subsequent analysis compared the two lists yielding a score which reflected the relative accuracy of the perception.
The analysis proceeded by constructing a square matrix of scores calculated by comparing each perception against all targets in the given dataset. The properly matched trials (on the main diagonal of the matrix) were assigned a statistical merit (Z-score) by comparison with the distribution of off-diagonal, mismatched scores, which had sufficiently Gaussian characteristics to allow robust parametric tests. As the database accumulated, this procedure created an empirical background distribution of scores against which the actual performance could be compared, and ultimately allowed a precise score to be assigned to each trial in the PRP experiment.15
Time and Distance
Over the life of the program, more than two decades, a large database of 650 independent trials was generated. These included some trials where the agent and percipient worked at the same time. A somewhat larger number of trials were done with the percipient producing the binary descriptor list, sketches and descriptions some hours or days after the agent visited the scene. The largest portion of the trials were done in the precognitive mode – the percipient made a report with sketches and the binary list as much as two or three days before the target was selected and visited by the agent. This protocol provided an additional level of control in the experiment, including a strong defense against any reasonable criticisms arguing possible normal communication.
More important, the scores in precognitive trials turned out to be statistically indistinguishable from those in the other conditions. The regression of score level on the time difference between the agent and percipient activity is non-significant. Similarly, the PRP experiment explored the distance separating agent and percipient and determined that it also does not affect scoring. Trials over five or 5,000 kilometers yielded similar results.16
Other parameters were explored, including ex post facto vs. participant-encoded descriptions, agent-chosen versus randomly-assigned targets, and single vs. multiple percipients. Most of these factors were not strong modulators of the scoring. These findings help delimit the kinds of models which have potential to explain how remote perception works. While the empirical status seems quite clear, explanations remain speculative in large part. The time and distance independence of the results are helpful qualities in a search for appropriate descriptions. They suggest something akin to nonlocality in quantum physics.
Variations
The remote perception program explored various scoring procedures in addition to the binary descriptors. One alternative added a third category allowing the participants somewhat more subtle distinctions. This was carried further to employ a nine-point scale providing still more apparent precision. Over time, the level of scoring decreased, suggesting that the efforts to give participants greater choice and more latitude for accurate descriptions were actually counterproductive. Brenda Dunne, the lead investigator for PRP, concluded that these explorations tended to encourage the intellectual over the aesthetic and free flowing style of thinking which characterized the early work in the paradigm. Even with the moderating effects of such explorations, the bottom line of the PEAR Remote Perception research program is strong. The composite probability across roughly 650 trials is about 3 x 10-10 corresponding to a six-sigma deviation.17
Theory and Models
PEAR's empirical results in technically sophisticated, controlled experiments over many years showed a need for some form of expansion or modification of the scientific models which have served well as descriptions of the world. A major exception in these otherwise competent models is that mind or consciousness have had no place, even though there is no question about their presence and importance in the world. Only in the past two or three decades have mainstream scientists undertaken to explain the sources and nature of consciousness.
The PEAR research program, together with similar research endeavors around the world, adds a further dimension to the challenge of describing consciousness. Nearly three decades of experiments demonstrated and documented anomalous physical phenomena that were significantly correlated with such subjective variables as intention, meaning, and resonance. The results were in stark contrast with established physical and psychological principles, but there were no well-developed theories in the field. Based on the PEAR lab experience, Jahn and Dunne sought to develop competent theoretical models or valid extensions of accepted theories. Several factors provided both justification and some guidance for extended models.
The anomalous results depend on operator intention and emotional resonance with the task, and they also show suggestive operator-specific structure in the data. PEAR looked for but did not find evidence of traditional learning or improvement with practice. Instead, operator debriefing interviews indicated that successful performance was more dependent on ‘getting out of the way’ and accepting that mind-machine interactions are possible. The finding that results had no explicit space or time dependence made explanations based on existing physical or psychological frameworks insufficient, leading to theoretical efforts at PEAR giving consciousness a proactive role in the establishment of its experience of the physical world.
Jahn and Dunne produced a series of progressively refined efforts beginning with a paper called ‘On the Quantum Mechanics of Consciousness, With Application to Anomalous Phenomena,’18 and later published with supporting materials in a book documenting the PEAR research, Margins of Reality.19
This and subsequent variants attempt a functional application of metaphors from physics to phenomena of mind. The major premise is that ‘the basic processes by which consciousness exchanges information with its environment, orders that information, and interprets it, also enable it to bias probabilistic systems and thereby to avail itself of some control over its reality. This model regards the concepts that underlie all physical models of reality, particularly those of observational quantum mechanics such as the principles of uncertainty, complementarity, exclusion, indistinguishability, and wave mechanical resonance, as fundamental characteristics of consciousness rather than as intrinsic features of an objective physical environment.’20
The next step toward a practical model recognized that both mental and physical qualities exist on a continuum from tangible to intangible or ephemeral, and suggested that there is a deep unconscious and intangible area where the mental and physical may intermix. A conscious thought or intention rests on an unconscious foundation, and similarly, a tangible material system has a corresponding intangible aspect. This recognition undergirds a proposal that the inherently probabilistic nature of unconscious mind and intangible physical mechanisms could be invoked to achieve anomalous acquisition of information about, or anomalous influence upon, otherwise inaccessible material processes. The model's viability depends on a workable representation of the ‘merging of mental and material dimensions into indistinguishability at their deepest levels’.21
Criticism
Although it was a well-supported research program at a major university, the PEAR lab was not insulated from criticisms and attacks by sceptics. Many of these were uninformed expressions of bias, but some were by serious scholars, and the PEAR group welcomed informed critiques which could be addressed and incorporated to improve the quality of research.
As is the case for virtually all controversial scientific topics, the Wikipedia entry for PEAR has little information about the research, but is comprised almost exclusively of sceptical statements and references. These are typically not supported by direct experience or knowledge of the experiments, but rely mainly on magazine articles and sceptics’ blog posts as sources.
Some professional sceptics did comment on PEAR. For example James Alcock mentioned various problems with the PEAR experiments such as poor controls and documentation with the possibility of fraud, data selection and optional stopping not being ruled out. He provided no documentation for any of these suspicions, but nevertheless concluded there was no reason to believe the results were from paranormal origin.22
A sceptic who built his career criticizing JB Rhine, the psychologist CEM Hansel, evaluated Jahn's early psychokinesis experiments at the PEAR laboratory, and, according to the Wikipedia article, wrote that a satisfactory control series had not been employed, that they had not been independently replicated, and that the reports lacked detail. Hansel noted that ‘very little information is provided about the design of the experiment, the subjects, or the procedure adopted. Details are not given about the subjects, the times they were tested, or the precise conditions under which they were tested.’ All of this is belied by the prolific output of publications and technical reports from PEAR.
Unfortunately, most of the critical views expressed about PEAR tend to be simple expressions of bias and an unquestioned belief in the standard models of science. For example, physics professor Milton Rothman is quoted as saying that Jahn's experiments at PEAR started from an idealistic assumption, ignored the laws of physics and had no basis in reality,23 but he provided no evidence for his belief nor indications that he had read any PEAR reports. A telling anecdote illustrates the point. The editor of a prominent scientific journal once told the lab’s founder and senior scientist, Robert Jahn, that he might consider publishing one of Jahn’s recent papers, provided the author would transmit it telepathically.
In a few cases, critical scientists put in the time and effort to replicate or emulate PEAR experiments and failed to confirm their results. A good example is Stanley Jeffers, who visited and consulted PEAR researchers while developing an optical interference experiment in which he obtained null results. He donated the equipment to the PEAR lab, and interestingly, in that context, the experiment showed a nominally significant effect.24
PEAR's speculative explanation for the difference is that Jeffers approached the experiment as a physics question without consideration of human factors such as motivation and comfort. His laboratory environment was sterile and the procedures were those customary in physics research. When the PEAR lab took over the experiment, it became a combination of 'the white turban and the white lab coat' -- in other words, the human participants and the the dual-slit technology were treated as equally important. More recently, a series of experiments by Dean Radin has yielded robust evidence (4.36 sigma) that the optical interference experiment is replicable.25
Legacy
In 2007, the PEAR lab formally closed its doors, having pursued a scientific assessment of mind-machine interaction and anomalous information transfer for some 27 years. One of the ongoing efforts over that period was communication of information and support for independent replications. The lab had an ongoing program of internships, including students from Princeton University and from many other schools. A number of formal research programs in other institutions such as the University of Giessen, Germany, and the Institute für Grenzgebiete der Psychologie und Parapsychologie in Freiburg, Germany were direct offshoots from PEAR.
The independent Global Consciousness Project (GCP) founded by PEAR's research coordinator, Roger Nelson, employed REG technology and an extended version of protocols developed for the FieldREG research program. PEAR technology formed the basis for a company called Psyleron, Inc. which is producing line of state-of-the-art REG equipment for both research and personal applications. Another developing venture arising out of the PEAR and GCP technology is ‘Entangled, a Conciousness App’ which promises a hardware-based random source and software for personal and global network monitoring of consciousness effects for mobile devices.
Most directly, the PEAR legacy includes the International Consciousness Research Laboratories (ICRL), which hosts a communication network and continuing research program as well as a publication house focused on the issues that were central to the PEAR laboratory.
Documentary
StripMindMedia (2006). The PEAR Proposition: A Quarter Century of Princeton Engineering Anomalies Research. Directed by Aaron Michels (520 minutes, 2 DVD discs, 1 Audio CD)
Roger D Nelson
Literature
Alcock, J. (2003). Give the null hypothesis a chance: Reasons to remain doubtful about the existence of psi. Journal of Consciousness Studies 10, 29-50.
Dobyns, Y.H., Dunne, B.J., Jahn, R.G., & Nelson, R.D. (2004). The MegaREG Experiment: Replication and interpretation. Journal of Scientific Exploration 18/3, 369-97.
Dunne, B.J., Dobyns, Y.H., & Intner, S.M. (1989). Precognitive remote perception, III: Complete binary database with analytical refinements. PEAR Technical Report 89002, Princeton Engineering Anomalies Research, Princeton Univ. School of Engineering/Applied Science.
Dunne, B.J., Nelson, R.D., & Jahn, R.G. (1988). Operator-related anomalies in a random mechanical cascade. Journal of Scientific Exploration 2/2, 155-79.
Dunne, B.J., & Jahn, R.G. (1992). Experiments in remote human/machine interaction. Journal of Scientific Exploration 6/4, 311-32.
Dunne, B.J., & Jahn, R.G. (2003). Information and uncertainty in remote perception research. Journal of Scientific Exploration 17/2, 207-41.
Ibison, M., & Jeffers, S. (1998). A double-slit diffraction experiment to investigate claims of consciousness-related anomalies. Journal of Scientific Exploration 12/4, 543-50.
Jahn, R.G. (1982). The persistent paradox of psychic phenomena: An engineering perspective. Proceedings IEEE 70/2, 136-70.
Jahn, R.G., Dunne, B.J., & Jahn, E.G. (1980). Analytical judging procedure for remote perception experiments. The Journal of Parapsychology 44/3, 207.
Jahn, R.G., & Dunne, B.J. (1986). On the quantum mechanics of consciousness, with application to anomalous phenomena. Foundations of Physics 16/8, 721-72.
Jahn, R.G., & Dunne, B.J. (1988). Margins of Reality: The Role of Consciousness in the Physical World. New York: Harcourt Brace.
Jahn, R.G. et al. (2000). Mind/Machine Interaction Consortium: PortREG Replication Experiments. Journal of Scientific Exploration 14/4, 499-555.
Jahn, R.G., & Dunne, B.J. (2001). A modular model of mind/matter manifestations (M5). Journal of Scientific Exploration 15/3, 299-329.
Jahn, R.G., Dunne, B.J., Nelson, R.D., Dobyns, Y.H., & Bradish, G.J. (2007). Correlations of random binary sequences with pre-stated operator intention: A review of a 12-year program. Explore: The Journal of Science and Healing 3/3, 244-53.
Jahn, R.G., & Dunne, B.J. (2011). Consciousness and the Source of Reality. ICRL Press.
Nelson, R.D., Ziemelis, U.O., & Cook, I.A. (1992). A microelectronic chip experiment: Effects of operator intention on error rates. Technical Note PEAR 92003, Princeton Engineering Anomalies Research, Princeton Univ. School of Engineering/Applied Science.
Nelson, R.D., Bradish, G.J., Jahn, R.G., & Dunne, B.J. (1994). A linear pendulum experiment: Effects of operator intention on damping rate. Journal of Scientific Exploration 8/4, 471-89.
Nelson, R.D., Bradish, G.J., Dobyns, Y.H., Dunne, B.J., & Jahn, R.G. (1998). FieldREG II: Consciousness field effects: Replications and explorations. Journal of Scientific Exploration 12/3, 425-54.
Nelson, R.D., Jahn, R.G., Dobyns, Y.H., & Dunne, B.J. (2000). Contributions to variance in REG experiments: ANOVA models and specialized subsidiary analyses. Journal of Scientific Exploration 14/1, 473-89.
Nelson, R.D. (2006). Time-normalized yield: A natural unit for effect size in anomalies experiments. Journal of Scientific Exploration 20/2, 177-99.
Radin, D.I., & Nelson, R.D. (1989). Evidence for consciousness-related anomalies in random physical systems. Foundations of Physics 19/12, 1,499-1,514.
Radin, D., Michel, L., Galdamez, K., Wendland, P., Rickenbach, R., & Delorme, A. (2012). Consciouness and the douple-slit interference pattern: Six experiments. Physics Essays 25/2.
Rothman, M.A. (1992). The Science Gap: Dispelling the Myths and Understanding the Reality of Science. Buffalo, New York, USA: Prometheus Books.
Schmidt, H. (1971). Mental influence on random events. New Scientist and Science Journal (June), 757-58.
Tart, C., Puthoff, H., & Targ, R. (1980). Information transmission in remote viewing experiments. Nature 284, 191.
Endnotes
- 1. Schmidt (1971).
- 2. Jahn (1982).
- 3. Jahn et al.(2007).
- 4. Jahn et al. (2000).
- 5. Radin & Nelson (1989).
- 6. Nelson et al. (1998).
- 7. Nelson et al. (1992).
- 8. Dunne et al. (1988).
- 9. Nelson et al. (1994).
- 10. Nelson (2006).
- 11. Nelson et al. (2000).
- 12. Dobyns et al. (2004).
- 13. Dunne & Jahn (1992).
- 14. Tart, Puthoff, & Targ (1980).
- 15. Jahn, Dunne, & Jahn (1980).
- 16. Dunne, Dobyns, & Intner (1989).
- 17. Dunne & Jahn (2003).
- 18. Jahn & Dunne (1986).
- 19. Jahn & Dunne (1988).
- 20. Jahn & Dunne (2001).
- 21. Jahn & Dunne (2001).
- 22. Alcock (2003).
- 23. Rothman (1992).
- 24. Ibison & Jeffers (1998).
- 25. Radin et al. (2012).